Cactoblastis cactorum
Life
> Eukaryotes >
Opisthokonta
>
Metazoa (animals) > Bilateria >
Ecdysozoa > Panarthropoda > Tritocerebra > Phylum:
Arthopoda > Mandibulata >
Atelocerata > Panhexapoda >
Hexapoda
> Insecta (insects) > Dicondyla > Pterygota >
Metapterygota > Neoptera > Eumetabola > Holometabola > Panorpida >
Amphiesmenoptera > Lepidoptera (moths and butterflies)
> Glossata > Coelolepida > Myoglossata > Neolepidoptera > Heteroneura >
Ditrysia > Apoditrysia > Obtectomera > Pyraloidea >
Family: Pyralidae > Subfamily:
Phycitinae > Genus: Cactoblastis
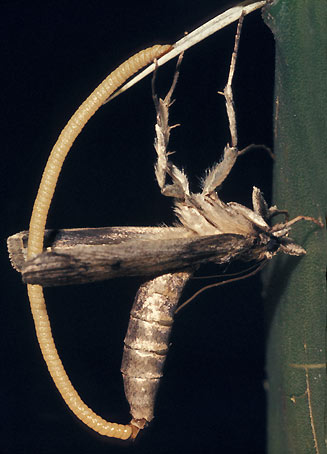 |
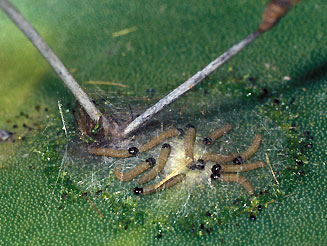 |
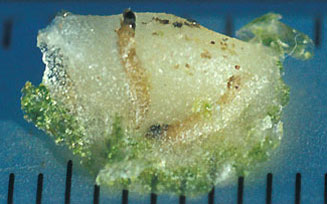 |
Figure 1a. Cactoblastis cactorum female laying
eggstick. [photo H. Roberson ©] |
Figure 1b (top). Cactoblastis cactorum first instar
larvae penetrating cladode surface, at base of spine (note base of
eggstick at top right).Figure 1c (bottom): First instar larvae embedded in gum. Gum
is a hazard for them when they first penetrate the cladode surface. [photos
H. Roberson ©] |
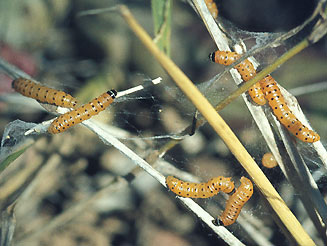 |
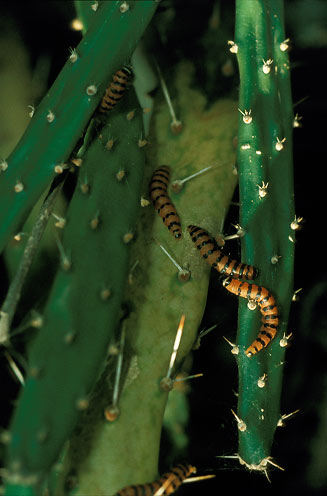 |
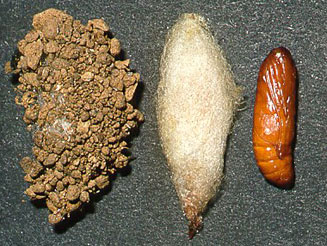 |
Figure 2a (top). Cactoblastis cactorum 3rd or 4th
instar larvae in search of a new cactus cladode to feed in, having eaten
out the previous one. Note the silken path they have constructed for
their journey. [photo H. Roberson ©]
Figure 2b (bottom). Cactoblastis cactorium cocoon with soil particles
adhering to it (left), cocoon without soil (middle), and pupa that has
been removed from the cocoon (right). [photo H. Roberson ©] |
Figure 2c. Cactoblastis cactorum final instar larvae
on Opuntia aurantiaca (Jointed cactus), either seeking out a new
cladode to feed in or about to find a place in the leaf litter to
pupate. The cladode in the centre is pale-coloured because most of the
tissue inside has been eaten by the larvae. [photo H. Roberson ©] |
Distribution and habitat
Native to northern Argentina, Uruguay, Paraguay
and southern Brazil. In addition, it has been introduced to the
following regions for the biological control of Opuntia
cactus weeds (Zimmermann et al. 2004):
-
Australia (introduced 1926, from Argentina)
-
New Caledonia (introduced 1933, from
Australia)
-
South Africa (introduced 1933, from
Australia)
-
Mauritius (introduced 1950, from South
Africa)
-
Hawaii (introduced 1950, from Australia)
-
Nevis in the Leeward Islands, Caribbean (introduced 1957, from
South Africa)
-
Antigua in the Leeward Islands, Caribbean
(introduced 1960, from Nevis)
-
Kenya (introduced 1966, from Antigua;
establishment unconfirmed)
-
Cayman Islands, Caribbean (introduced 1970,
from Nevis and Antigua)
-
St Helena, mid-South Antlantic (introduced
1971, from Nevis and Antigua)
-
Ascension Island, mid-South Atlantic
(introduced 1973, from St Helena)
-
Pakistan (introduced 1994, from Australia;
establishment unconfirmed)
Cactoblastis cactorum has also become
established by accident in the USA (first recorded from Florida in 1989) either
through migration from the Carribean islands and/or importation on
ornamental cacti. This is a serious issue as it is attacking the
indigenous cacti in the region (Zimmermann et al. 2000).
Life cycle
Adults
Cactoblasis cactorum adults emerge from
pupae in the evening, usually within two hours after dusk. Calling
and mating takes place mainly in the period of about an hour and a
quarter before sunrise (Hight et al. 2003 - USA study).
Females adopt a calling posture with the abdomen protruding upwards
through the wings, at an angle of 45 degrees and for those that
ultimately are successful in mating, the calling period only lasts
for about five minutes before a male arrives and mates. Unsuccessful
females call for a lot longer (about 40 minutes). Calling involves
releasing a sex pheromone that attracts a male that is downwind from
the female. The copulation period lasts for an average of 32 minutes
(Hight et al. 2003).
During the day, adults usually remain inactive
and sit motionless in the vegetation in the vicinity of their host
plants. Very rarely, if ever, do they shelter on the host plant
itself (I searched for eggsticks daily on hundreds of plants in the
field over summer and winter egg laying periods without once seeing
adults on the host plants). Oviposition (egg laying), like
emergence, usually occurs within two hours after dusk (Dodd 1940 p.
122 - Australia) although oviposition can continue until about
midnight (Pettey 1948 p. 42).
Adults do not feed and therefore have to rely
on energy stores accumulated in the larval stage. Hence, they have a
short life, surviving for about five or six days although at lower
temperatures (18șC) they can survive for about 12 days (Legaspi and
Legaspi 2007; Legaspi et al. 2009).
The dispersal capability of adults C.
cactorum has become an important issue in developing a strategy
for controlling its spread in the USA (Sarvary et al. 2008).
Myers et al. (1981) found that among a group of plants, those
that had C. cactorum moths placed on them before evening
commenced, were more likely to have eggsticks laid on them than
those which did not. Similarly, plants attacked in the previous
generation were more likely to have eggsticks laid on them in the
following generation (Dodd 1940 p. 121; Myers et al. 1981;
Robertson 1985a, 1987), suggesting that moths emerging from
pupae in the vicinity laid at least some of their eggs near the
emergence site (although one can't discount the possibility that
these plants were inherently more attractive to ovipositing
females).
Although there is abundant evidence of C.
cactorum dispersing very little, there are also a number of
records of long distance dispersal by C. cactorum females.
Dodd (1940 p. 121) remarks "many instances on record indicate that
individuals have flown as far as fifteen miles to oviposit". In the
Leeward Islands, C. cactorum appears to have dispersed
naturally from some of the islands to others. For instance, it
colonised the island of St Kitts from Nevis four miles away (Garcia
Tuduri et al. 1971). Similarly, in the Hawaiian Island chain,
C. cactorum has apparently dispersed naturally through these
islands over a period of about seven years (C.J. Davis in Garcia
Tuduri et al. 1971).
On the basis of the above evidence for short
and long distance dispersal in C. cactorum, and as proposed
by Osmond and Monro (1981), it is likely that the dispersal
behaviour of C. cactorum females is age-related, with females
laying their first eggstick(s) near the emergence site and then
dispersing to lay the rest of their egg complement further afield.
Osmond and Monro (1981) suggest that oviposition of the eggs near
the emergence site by C. cactorum "probably acts as an
insurance against flying into regions devoid of prickly-pear and
thus dying without finding a host". Another possible reason for
oviposition prior to dispersal is to reduce the energy cost of
flight. In C. cactorum, a full egg complement constitutes
about 46% of female mass (Robertson 1985a) and thus the tendency may
be to reduce wing-loading by laying part of the egg complement
before dispersal. This type of behaviour is commonly recorded in
moths that emerge gravid from the pupa (Johnson 1969 pp. 181-182;
Baker 1978). Puzzlingly, Sarvary et al. (2008) in their study
of flight performance of C. cactorum considered unmated
versus mated males and females but do not appear to have considered
the effect of the reduction of egg load on flight performance.
Eggs and egg laying
The most distinctive feature of C. cactorum
biology is the clumping of the eggs in eggsticks (Figure 1a).
The number of eggs per eggstick typically ranges from 4 to 111 eggs,
averaging about 56 eggs (Robertson 1985a). Dodd (1940) and Pettey
(1948) recorded averages higher than this, ranging from 68 to 96 but
I suspect there was a bias to the larger eggsticks. They each
independently recorded a maximum number of 150 eggs per eggstick.
The laying of an eggstick (Figure 1a)
involves first depositing an amber-coloured substance near the tip
of the selected cactus spine which is used to glue the first egg to
the spine. The positioning of eggs on top of one another is guided by
setae that surround the ovipositor. Each egg is pressed against the
last egg in the eggstick. According to Pettey (1948 p. 41), eggs are
laid at an average rate of one every 16 seconds (fastest: 1 every 10
seconds; slowest: 1 every 24 seconds) so that the average 56 egg eggstick
takes about 9-22 minutes to lay.
Under near optimal temperature conditions,
fecundity (number of eggs laid by each female) varies from 54 to
281 eggs (Robertson 1985a). Each female usually lays 3 to 4
eggsticks (range 2-7). Fecundity is detrimentally affected by low
temperatures (Robertson 1989), such that, in the temperature range
from 8 - 20șC there is a linear relationship between the mean
number of eggs laid per female and the mean minimum temperature at
the time of egg laying. Under cold conditions females often fail to
lay all the eggs inside them.
In the Eastern Cape, South Africa, there are
typically
two generations a year: At a study site on Thursford Farm, 21 km NW
of Grahamstown (Robertson 1985a), the egg laying period
in the summer
generation lasted from the beginning of October
through to mid-December and by the end of January they had all
hatched. Winter generation eggs were laid from the beginning of
March to early May. Because of the cooling temperatures, late laid
winter eggs took a long time to hatch so it was only by mid-August that
they had all hatched. Egg laying in other parts of the Eastern Cape can start as
early as September in the summer generation and February in the
winter generation.
In the Thursford study, the egg development
period lasted an average of 51 days in the summer generation
(range: 41-64) and 75 days in the winter generation (range 58-114).
Pettey (1948 p. 64) recorded much shorter average development
periods at other localities in the Eastern Cape (e.g. 33 days in
summer generation and 50 days in winter generation at
Graaff-Reinet), and Dodd (1940 p. 117) recorded even shorter
development periods in Australia (28-30 days in summer generation
and 23-38 days in winter generation in Southern Queensland).
Temperature has a direct effect on egg development: development
halts altogether at about 12șC and reaches its maximum rate at about
30șC (Robertson 1985a, Appendix 4; Legaspi and Legaspi 2007). By
35șC, the egg development rate has dropped off slightly. Under
constant near optimal temperature conditions in the laboratory
(30șC), the minimum egg development time was about 20 days (Legaspi
and Legaspi 2007) although Dodd (1940) recorded a minimum period of
18 days.
Larvae
The larvae that hatch from an eggstick
penetrate the plant together (Figure 1b). The first larvae that
emerge move down to the base of the spine where they surround
themselves with a web of silk spun between the spine and the cladode
surface, perhaps as a way of protecting them from predators such as
ants. When the number of larvae reaches a certain undetermined
threshold level, the larvae begin penetrating, usually adjacent to
the spine on which the eggstick was laid. As few as nine larvae are
able to successfully penetrate a cladode. To penetrate, the larvae
chew away the cuticle at one spot on the cladode surface and deposit
it in a ring around the penetration site (Figure 1b). If penetration
is prevented by a tough cuticle or because of gum exudation (Figure
1c), the surviving larvae usually attempt to penetrate elsewhere on the plant.
After successful penetration, the larvae feed
gregariously on the parenchymous tissue inside the cladode. Fibres
are not eaten. Larvae can tunnel from cladode to cladode thus
avoiding having to move outside and start penetrating the plant
elsewhere. However, larvae sometimes do have to vacate the cladode
when:
-
the cladode becomes detached from the plant
and contains no more suitable tissue for consumption;
-
they destroy the entire plant in which they
are feeding;
-
they are unable or unwilling to bore into
adjacent cladodes, particularly when the adjacent cladode is
woody;
-
the internal cladode temperature is too
high. Under the latter circumstances, larvae, when outside the
cladode, sometimes suspend themselves on a web of silk that is
spun in the shade beneath a cladode.
When locating a new penetration site, larvae
spin a silken path (Figure 2a), which presumably maintains colony
cohesion as well as enabling the larvae to retrace their route if
necessary. After about the third instar, larvae in some colonies
split up into smaller groups, especially when the cladodes are
small.
Larvae usually pass through six instars (each
instar is separated by a moult of the old cuticle) although there
can be as many as eight (Robertson 1985a). As larvae get bigger, they develop vivid
orange and black banding, which is believed to act as a warning to
predators that they are distasteful (such colouring is termed
aposematic colouration).
Regarding the phenology of larvae (i.e.
the times of the year when they were present), at the Thursford site
in the Eastern Cape, the summer generation larvae were present from near the beginning of December
through to mid-March
although there were a few colonies that skipped a winter generation and passed
right through winter, only pupating in August. Winter generation
larvae were present from the beginning of April through to the
beginning of November.
In the Thursford study, the larval development period
ranged from 46 to 53 days in summer (excluding the overwintering
larvae) and 90 to 141 days in winter. The larval development period
of the overwintering summer generation larvae was 200-210 days.
Pettey (1948) at Uitenhage and Graaff-Reinet in the Eastern Cape,
recorded larval development periods ranging from 47-82 days in
summer and 99 to 164 days in winter.
Pupae
When larvae reach maturity they move to the
ground where they spin a cocoon in a secluded place,
typically among the leaf litter or in loose soil. Within the cocoon
the larva moults its skin to reveal the pupal form beneath. Within
the pupa, major redevelopment happens with genes for adult
characters such as wings being turned on and genes for larval
characters being turned off.
In the study by Pettey (1948 pp. 61, 65), the
pupal development period ranged from 20 to 32 days in the
summer generation and 56 to 86 days in the winter generation.
Ecological interactions in southern Africa
Larval host plants
Unless otherwise indicated, information is from
Zimmermann et al. (2000) and Zimmermann et al. (2004).
The comments about impact are from a biological control perspective,
in terms of how effective the larvae are in destroying the plant.
-
Opuntia aurantiaca (Jointed cactus). Moderate to
significant impact, mainly on large plants.
-
Nopalea cochenillifera (= Opuntia cochenellifera)
-
Opuntia engelmannii. Moderate impact
-
Opuntia ficus-indica (Prickly pear). Ineffective in
killing large woody plants but highly effective in destroying
small plants.
-
Opuntia humifusa. Significant impact.
-
Opuntia monacantha. Has a moderate to significant
impact.
- Opuntia pusilla. Cultivated in South Africa. Recorded
as being attacked by Cactoblastis cactorum in Florida,
USA.
-
Opuntia robusta. Has a significant impact, especially
damaging to small plants. Cactoblastis cactorum is a pest
of the cultivated spineless varieties of this species.
-
Opuntia salmiana. Moderate impact, mainly on larger
plants.
-
Opuntia spinulifera
-
Opuntia stricta. This is the species that
Cactoblastis cactorum decimated in Australia but in South
Africa, it has been less effective.
- Opuntia tomentosa. Cultivated in southern Africa. Has an insignificant impact.
-
Cylindropuntia imbricata. It does not thrive on
this plant
Predators
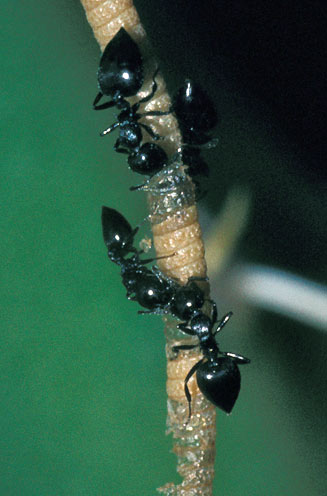 |
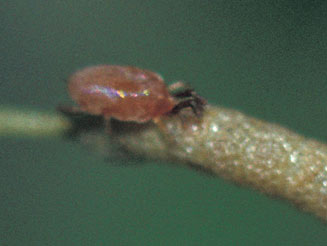 |
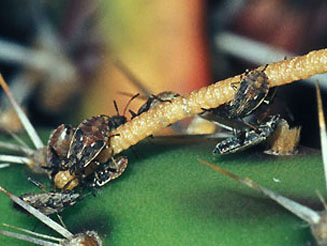 |
Figure 3a. Crematogaster liengmei ants eating eggs in
eggstick of Cactoblastis cactorum. [photo H. Roberson ©] |
Figure 3b (top). Unidentified mite extracting the contents of
an egg in an eggstick of Cactoblastis cactorum. [photo H. Roberson ©]
Figure 3c (bottom). Nysius bugs (Lygaeidae) eating eggs
in eggstick of Cactoblastis cactorum. [photo H. Roberson ©] |
Eggs are eaten by:
- ants (Hymenoptera: Formicidae). Egg predation by ants varied
from 55-77% % at a site 22 km NW of Grahamstown
(Robertson 1985a, 1985b, 1988; Robertson and Hoffmann 1989). Ant
taxa attacking eggs included:
- Crematogaster liengmei
- Pheidole sp.
- Tetramorium erectum
- Monomorium albopilosum
- Monomorium sp. (monomorium-group)
- Camponotus niveosetosus
Pettey (1948 p. 78) recorded the ant
Technomyrmex albipes feeding on C. cactorum eggs,
although this ant is almost certainly Technomyrmex pallipes
as, until a recent revision of the genus, T. albipes
was confused with T. pallipes in southern Africa.
Ants are attracted on to
Opuntia ficus-indica,
Opuntia aurantiaca,
and probably other opuntias, by extrafloral nectaries situated
at the base of areoles (from where the spines eminate).
- Nysius (Hemiptera: Lygaeidae). Of minor importance in comparison
to ants (Robertson 1985a; Robertson and Hoffmann 1989).
- mites (Arachnida: Acari). Of minor importance in comparison
to ants (Robertson 1985a; Robertson and Hoffmann 1989)..
Larvae are eaten by:
- ants (Hymenoptera: Formicidae).
- Anoplolepis steingroeveri (Robertson 1985a;
Robertson and Hoffmann 1989).
- Pheidole sp. (Robertson 1985a; Robertson and
Hoffmann 1989).
Pupae are eaten by:
- ants (Hymenoptera: Formicidae)
- Dorylus helvolus (a mainly subterranean driver
ant). Robertson (xxx) recorded xx% mortality of pupae by
these ants.
Parasitoids
Eggs are parasitised by:
Larvae are parasitised by:
- Pseudoperichaeta sp. (Diptera: Tachinidae). An
incidental parasitoid of C. cactorum larvae (Robertson
1985a; Robertson and Hoffmann 1989).
Pupae are parasitised by:
- Hymenoptera > Chalcididae
- Brachymera sp. (Pettey 1948)
- Invreia sp. (Robertson 1985a; Robertson and Hoffmann
1989)
- Euchalcidia sp. (Robertson 1985a; Robertson and
Hoffmann 1989)
Diseases
-
Nosema cactoblastis (Fungi:
Microsporidia: Nosematidae). Rarely encountered in the field
(Pemberton and Cordo 2001). In my experience in the field in
the Eastern Cape, South Africa, I only ever once found a
number of colonies in the same area that had died of a
disease, possibly Nosema.
History of biological control in Australia and
South Africa
The first use of
Cactoblastis cactorum for biological control was in
Australia against the prickly pear Opuntia stricta (Dodd
1936, 1940, 1959) and is one of the world's outstanding examples
of the successful biological control of a serious weed problem.
Prior to the initial release of C. cactorum in 1926,
about 24 282 000 hectares of land in Australia were infested by
prickly pear, half of which were densely infested and
agriculturally useless. Mechanical and chemical methods of
control were not feasible, the cost of clearing a densely
infested area being between 6.7 and 40 times the value of the
land (calculated from Dodd 1940 p. 2). The use of biological
control had already been considered when in 1912 the Queensland
Prickly Pear Travelling Commission was appointed and sent to
various parts of the world where cacti occurred in an attempt to
find natural enemies that would attack O. stricta (Mann
1970). In 1914 one of the members of the commission discovered
C. cactorum in the Botanic Gardens at La Plata, Argentina
but the larvae he sent across to Australia died before pupation
(Dodd 1940 p. 108). The First World War interrupted events and
it was only after the appointment of the Commonwealth Prickly
Pear Board in December 1919 that more research on biological
control was instigated. From late 1920 through to 1937
entomologists working in the Americas discovered about 150
different species of natural enemies on Opuntia species
and related cacti, of which 50 were dispatched to Australia.
In 1925, 2750 eggs of C.
cactorum reached Australia. These eggs were derived from
larvae that were collected from Opuntia delaetiana and a
similar species at Concordia, Entre Rios in Argentina (Dodd 1940
p. 109). C. cactorum satisfied host specificity
requirements and in February-March 1926 eggs were first
released. This was the start of a massive campaign to distribute
C. cactorum eggs throughout the infested areas of
Queensland and New South Wales. An impressive total of
approximately 2750 million eggs (weighing a total of about
800-900 kg) were distributed between 1926 and 1931 by government
authorities (Dodd 1940 p. 115). By 1932, most of the prickly
pear plants were destroyed to ground level but were not
necessarily killed because they had woody cladodes below ground
level resistant to C. cactorum attack. During 1932 and
1933 the C. cactorum population was suddenly reduced in
size because the larvae had consumed most of the available food
supply. Consequently the plants that were not killed by C.
cactorum during the first wave of attack sent up a vigorous
regrowth. The population of C. cactorum recovered quickly
after this increase in food supply so that by 1935 the regrowth
was under control (Dodd 1940 p. 5). Repeated destruction of the
above-ground portions eventually resulted in the death of the
wood rooted cladodes. Waves of prickly pear resurgence and then
collapse continued but on a more localised level (Dodd 1940 p.
141). The end result was that in Queensland, complete biological
control of prickly pear had been achieved. Widespread
infestations of O. stricta had been reduced to isolated
or widely scattered plants with only small patches of resistant
prickly pear remaining. By 1940, 8 900 000 hectares of country
were open for reoccupation by farmers. The benefit to the
farmers was enormous but no statistics are available to show the
economic recovery brought about by this biological control
programme (Dodd 1959 p. 576). In New South Wales C. cactorum
was not as successful as in Queensland but infestations were
nevertheless reduced by at least 80% (Dodd 1940 p. 8).
In South Africa, C.
cactorum was first released in 1933 against Opuntia
ficus-indica from material derived from the Australian
population. From 1933 to 1941 about 579 million eggs were
distributed over an infested area in the Eastern Cape totalling
about 598 300 hectares (Pettey 1948 p. 31). The effectiveness of
C. cactorum on O. ficus-indica was not nearly as
great as it was in Australia on O. stricta. The
difference in performance was attributed mainly to the more
tree-like and woody habit of O. ficus-indica. C. cactorum
was effective in destroying young plants (below a height of
about 0.6 m) but with older plants only the first two or three
terminal cladodes were eaten so that the woody stumps still
remained. The latter still had a great capacity for producing
regrowth. Pettey, in a report he made after a visit to
Australia, had in fact predicted this state of events before
C. cactorum was released in South Africa (Pettey 1948 p.
15).
Subsequent research I
undertook in the 1980's (Robertson 1985a, b, 1989; Robertson and
Hoffmann 1989) showed that predation by ants and temperature
effects on fecundity were also partly responsible for the
relatively poor performance of C. cactorum in South
Africa. My research also included a comparison of the
effectiveness of C. cactorum on Opuntia ficus-indica
(Prickly pear) and Opuntia aurantiaca (Jointed
cactus). Despite the difference in growth form between these two
host plants, regrowth from the rarely eaten woody cladodes was
an issue for both host plant species.
Publications
- Baker R.R. 1978. The Evolutionary
Ecology of Animal Migration. Hodder
& Stoughton, London.
- Dodd A.P. 1940. The biological
campaign against prickly-pear.
Commonwealth Prickly-pear Board
Bulletin. Government Printer, Brisbane.
- Foxcroft L.C., Hoffmann J.H.,
Viljoen J.J., Kotze J.J. 2007.
Factors influencing the distribution of
Cactoblastis cactorum, a biological
control agent of Opuntia stricta in
Kruger National Park, South Africa.
South African Journal of Botany 73(1):
113-117. doi:10.1016/j.sajb.2006.09.009
- Garcia Tuduri J.C., Martorell L.F.
and Gaud S.M. 1971. Geographical
distribution and host-plants of the
cactus moth, Cactoblastis cactorum
(Berg) in Puerto Rico and the United
States Virgin Islands (Lep.,
Phycitidae). Journal of Agriculture of
the University of Puerto Rico 55:
130-135.
- Hight S.D., Bloem S.,
Bloem K.A., Carpenter J.E. 2003. Cactoblastis cactorum
(Lepidoptera:
Pyralidae): observations of courtship
and mating behaviors at two locations on
the Gulf Coast of Florida. Florida
Entomologist 86(4): 400-408.
- Johnson C.G. 1969. Migration and
Dispersal of Insects by Flight.
Methuen, London.
- Legaspi J.C., Baez I.
nad Legaspi B.C. 2009. Reproduction,
longevity, and survival of Cactoblastis cactorum (Lepidoptera:
Pyralidae). Annals of the Entomological
Society of America 102(3): 445-449.
- Legaspi J.C. and Legaspi
B.C. 2007. Life table analysis for Cactoblastis cactorum immatures and
female adults under five constant
temperatures: implications for pest
management. Annals of the Entomological
Society of America 100(4): 497-505.
- Mafokoane L.D., Zimmermann
H.G., Hill M.P. 2007. Development
of Cactoblastis cactorum (Berg)
(Lepidoptera : Pyralidae) on six North
American Opuntia species. African
Entomology 15(2): 295 - 299.
- Myers J.H., Monro J. and Murray N.
1981. Egg clumping, host plant selection
and population regulation in
Cactoblastis cactorum (lepidoptera).
Oecologia (Berlin) 51: 7-13.
- Osmond C.B. and Monro J. 1981.
Prickly pear. In: Plants and Man in
Australia. Eds D.J. Carr and S.M.
Carr. Academic Press, Sydney, pp.
194-222.
- Pemberton R.W. and Cordo H.A. 2001.
Nosema (Microsporida:
Nosematidae) species as potential
biological control agents of
Cactoblastis cactorum (Lepidoptera:
Pyralidae): surveys for the
microsporidia in Argentina and South
Africa. Florida Entomologist 84(4):
527-530.
- Pettey F.W. 1948. The biological
control of prickly pears in South
Africa. Union of South Africa,
Department of Agriculture, Science
Bulletin 271, (Entomology Series No.
22): 1-163.
- Robertson H.G. 1985a. The
ecology of Cactoblastis cactorum
(Berg) (Lepidoptera: Phycitidae) in
relation to its effectiveness as a
biological control agent of prickly pear
and jointed cactus in South Africa.
Unpublished PhD thesis, Rhodes
University, Grahamstown.
- Robertson H.G. 1985b. Egg
predation by ants as a partial
explanation of the difference in the
performance of Cactoblastis cactorum
on cactus weeds in South Africa and
Australia. Proceedings of the VI
International Symposium on the
Biological Control of Weeds, 19-25
August 1984, Vancouver, Canada (ed. E.S.
Delfosse), pp. 83-88.
- Robertson H.G. 1987.
Oviposition site selection in Cactoblastis cactorum (Lepidoptera):
constraints and compromises. Oecologia
73: 601-608.
- Robertson H.G. 1988.
Spatial and temporal patterns of
predation by ants on eggs of Cactoblastis cactorum. Ecological
Entomology 13: 207-214.
- Robertson H.G. 1989.
Seasonal temperature effects on
fecundity of Cactoblastis cactorum
(Berg) (Lepidoptera: Pyralidae):
differences between South Africa and
Australia. Journal of the Entomological
Society of Southern Africa 52: 71-80.
- Robertson H.G. &
Hoffmann J.H. 1989. Mortality and
life-tables of Cactoblastis cactorum
(Berg) (Lepidoptera: Pyralidae) compared
on two host-plant species. Bulletin of
Entomological Research 79: 7-17.
- Sarvary M.A., Bloem K.A., Bloem S.,
Carpenter J.E., Hight S.D. and Dorn S.
2008. Diel flight pattern and flight
performance of Cactoblastis cactorum
(Lepidoptera: Pyralidae) measured on a
flight mill: influence of age, gender,
mating status, and body size. Journal of
Economic Entomology 101(2): 314-324.
- Stange G. 1997. Effects
of changes in atmospheric carbon dioxide
on the location of hosts by the moth, Cactoblastis cactorum.
Oecologia 110(4): 539-545. DOI:
10.1007/s004420050192
- Stange G., Monro J.,
Stowe S. and Osmond C. B. 1995. The CO2
sense of the moth Cactoblastis
cactorum and its probable role in
the biological control of the CAM plant
Opuntia stricta. Oecologia
102(3): 341-352. DOI: 10.1007/BF00329801
- Zimmermann H.G., Moran V.C. and
Hoffmann J.H. 2000. The renowned cactus
moth, Cactoblastis cactorum: its
natural history and threat to native
Opuntia floras in Mexico and the
United States of America. Diversity and
Distributions 6: 259-269.
- Zimmermann H.G., Bloem S. and Klein
H. 2004. Biology, History, Threat,
Surveillance and Control of the Cactus
Moth, Cactoblastis cactorum.
IAEA, Vienna.
|
|
Text by Hamish Robertson
|